|
 |
|
 |
|
LIGHT GUIDING LIGHT
Clifford Patterson Lecture, Royal Society,
London
10 December 2001
I would like to begin by painting a picture. Imagine a
transparent cube of material (like the one in this slide
Fig 1) with a myriad of interconnections and components
are created by light itself. Circuits that lie on top of
each other. Circuits that are reconfigurable. Virtual Circuitry.
This is our dream. Light directs and manipulates light without
any intervening fabricated components.
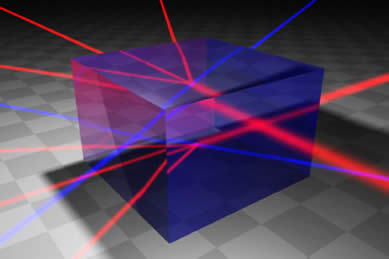
Ladies and Gentleman, I am greatly honoured to share these
ideas with you, especially on this particular day. Because,
10 December is a monumental date in history.
On this day, 100 years ago, Marconi transmitted the first
wireless signal across the Atlantic. We all know what that
historic day foreshadowed. But, what amazes me is that there
are possibly hundreds of people alive today who are old
enough to remember that celebrated event.
The technological advances that have transpired throughout
their lifespan have been nothing short of extraordinary.
There have even been extraordinary advances in telecommunications
over the last 35 years. That's when I first arrived here
in London to research light transmission along optical fibres.
Though, strange as it may seem, not from a background of
optical communications, but rather from my interests on
visual photoreceptors of animal eyes like the ones shown
here.
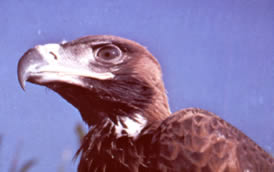
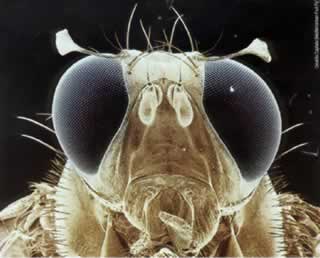
Now today, optical fibre telecommunications is the worldwide
standard. But, back in 1967 experts argued that it was an
unnecessary technology and worse still, it was infeasible
due to the insurmountable problem of glass absorption losses.
Yet only a few years later, previously unimaginable amounts
of information were being transmitted across the Atlantic
by optical fibres. In many ways, the situation regarding
the feasibility of light guiding light technologies are
analogous to the state of optical fibre telecommunications
back in 1967.
Today, like in 1967 many experts proclaim that we don't
need such a technology and that it is unlikely that we will
ever surmount problems with materials. I want to emphasise
up front that, unlike the experts of 1967, these present
day sceptics may very well be right.
Nevertheless, the concepts of this futuristic technology
are so beautiful and the conceivable devices so alluring,
that I am compelled to hope that this technology will one
day be a reality - devices and maybe even toys that implement
light guiding light concepts.
But, I must stress from the outset that I will tonight
discuss only the concepts behind the technology and not
the technology itself nor the impediments to it. So let
us begin this futuristic journey - a journey which could
be the impetus for one of you to make this technology a
reality.
As I have said, the building blocks for light guiding light
are free-standing beams, known as spatial solitons. Unlike
the usual light beams, which spread or diffract, solitons
create their own channel as they travel in a uniform medium,
remaining localized and preserving their shape. To produce
a soliton, you need a material whose refractive index changes
with the beam's brightness
Normally beams do not influence each other. But solitons
can attract, repel and spiral around each other and this
interaction can even be described by the classical force
laws treating the beams as particles with mass.
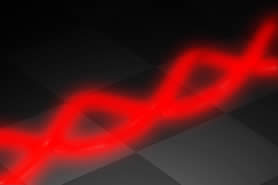
Whereas linear waves always pass through one another, solitons
can be dramatically altered by collisions. They can annihilate
one another, fuse (Fig. 5) or give birth to multiple solitons.
These phenomena turn out to be of fundamental importance
to the emerging technology of light guiding light and light
written circuitry.
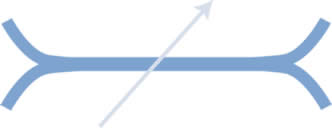
I want to emphasise that the story of manipulating light
with light to create virtual circuitry is not just about
an emerging technology. Research into this field has also
revealed new conceptual approaches for understanding how
curious light beams, known as optical spatial solitons,
can be made to remain localized in space while performing
some rather amazing acrobatics.
Advances of late have been remarkably swift, coming from
different groups across the globe:
-
Spatial solitons, once the domain of high power lasers,
can now be launched by an incandescent light bulb;
-
Soliton dynamics, once the province of esoteric mathematics,
is now accessible with undergraduate physics;
-
Mere theoretical predictions of a few years ago, such
as the possibility of one soliton being made to spiral
about another, the fusion or the creation of solitons
upon collision, and the transportation of a dim beam by
a bright beam are now readily observable in the laboratory.
|
I have been describing what solitons can do. But advances
in technology require an understanding of why they can do
it. Clearly, we need to have a physical understanding of
solitons. And, this at first posed a significant bottleneck,
because it seemed to necessitate intractable mathematics
of nonlinear physics.
But, today we now can appreciate solitons from elementary
physics. To set the stage, recall the physics of linear
optical waveguides. Optical beams have an innate tendency
to spread as they propagate in a homogeneous medium. However,
this beam diffraction can be compensated for by the lens
like action of beam refraction, if the refractive index
is increased in the region of the beam.
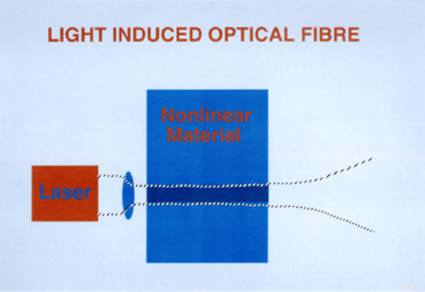
The resulting optical waveguide provides a balance between
diffraction and refraction. Spatial solitons can also be
understood from this familiar perspective.
Conceptually speaking, nonlinear beams interact with matter
to create their own waveguides (Fig 7). This occurs because
the refractive index of a nonlinear medium depends on the
physical properties of the light beam. We emphasise that
these induced waveguides are composed of linear material
and are of arbitrary shape, even twisted and contorted.
Beams then propagate along their own induced waveguide according
to the familiar physics of linear optics.
Of course, if you change the shape or form of the exciting
beams then you change the shape and form of the induced
waveguide. A simple self-consistency relationship exists
between the soliton and the modes of its induced waveguide.
A soliton is a mode or modes of the optical waveguide it
induces.
Armed with this elementary perspective, we can appreciate
that disparate types of solitons are actually the same animal.
In the simplest case, a soliton is one mode of the waveguide
it induces. This describes the classical optical soliton
of Chaio, Garmire and Townes but it also predicted more
esoteric solitons such as the so-called vortex solitons. |
More generally, a soliton can be two or more modes of its
induced waveguide. This elaboration predicted interesting
soliton dynamics, it also explains incoherent solitons,
it explains multi-humped solitons and the coexistence of
different classes of solitons.
If a soliton can be composed of a number of modes, each
travelling at a different speed, then it should be possible
to decompose the soliton into its constituent modes in exact
analogy to Newton's refraction of white light by a prism
into its component colours. And this elementary physical
picture foreshadows novelties such as symmetric soliton
beams being transformed into asymmetric beams upon colliding
with one another.
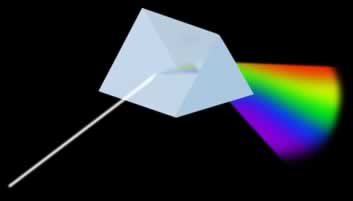
The fact that nonlinear propagation has a linear waveguide
equivalent provides a powerful conceptual tool, one that
guides us in a physical manner to the fundamental equations
and to their solutions. It allows us to predict novel phenomena,
motivate light written circuitry, and foreshadow the design
of lossless waveguide components as we discuss below.
Put simply, all soliton dynamics have a optical fibre analogue,
albeit some unusual shaped optical fibre system. Conversely
all phenomenon on optical fibres has its soliton equivalent
in some nonlinear medium. A self-consistency relation unites
the linear and nonlinear equivalents.
I cannot over state the importance of this theoretical
framework. Until its appearance, soliton science had a mystique
because it appeared to be divorced from our intuition -
an intuition that has been built upon linear phenomenon.
The linear perspective on solitons that I have presented
smashes this misconception. And, it facilitates unforeseen
generalisations and allows for numerous predictions, such
as vortex solitons, dynamic spiralling solitons, fusing
solitons.
For example, one major challenge is to find a simple analytical
description of solitons and their interactions. To achieve
this, it turns out that we need only borrow from the literature
of the linear harmonic oscillator. This is because every
optical fibre has a soliton equivalent. Therefore it is
natural to first consider the simplest optical fibre possible
- one whose refractive index falls off parabolically.
Light beams are described by the familiar harmonic oscillator
in this medium - one of the most basic equations of physics.
This elementary model tells us that Gaussian shaped beams
remain Gaussian shaped as they propagate. In general the
beams undulate periodically, undergoing periodic trajectories.
According to the above linear perspective on solitons,
Gaussian shaped solitons must also exist in some homogeneous
nonlinear medium with the same behaviour as beams in a parabolic
index optical waveguide composed of linear material. |
The particular nonlinear medium is found by using the self-consistency
relation. This leads to a medium whose nonlinear induced
refractive index change depends on the beam total power
only. This arises, for example, when the medium has a nonlocal
response with a correlation length that is much larger than
the beam diameter.
In such a medium, Gaussian shaped soliton beams remain
Gaussian and they are unaltered by colliding with one another.
For a special beam radius and power, a Gaussian beam will
propagate without change. Such a beam is called a stationary
soliton. It induces a graded index optical fiber which can
guide a signal beam. Apart from those beams with a special
radius, all other beams "breathe" as they propagate
with their radius oscillating periodically.
What happens to two stationary solitons that are initially
launched in parallel to each other?
In a homogeneous linear medium they would diffract as they
travel in a straight trajectory. In this nonlinear medium
they can attract and undergo periodic collisions with one
another or, if launched skew to each other, spiral about
each other as shown.
Finally, a distant "dim" beam can remain localized
and be guided and steered by a "bright" soliton
beam. Most experiments to date have involved comparatively
narrow solitons launched by a coherent source. These beams
induce waveguides that can propagate one or several modes.
At the other extreme it is possible to have comparatively
large incoherent soliton beams. And, in a beautiful experiment,
Mitchell and Segev have even launched them from an ordinary
incandescent light bulb.
Such "big" incoherent solitons can be very neatly
viewed as being composed of an enormous number of modes
of the multimoded waveguide they induce. This is a straightforward
application of the self-consistency principle.
But, diffuse light propagation along multimoded linear
waveguides can be described by classical geometric optics.
So again invoking the self-consistency principle, incoherent
solitons can also be viewed as bundles of rays, each ray
obeying the paraxial ray equation, or equivalently as a
bundle of classical (non-interacting) point particles, each
particle obeying Newton's laws of motion. This really is
extraordinary - solitons described by Newton's laws of motion
rather than the esoteric nonlinear Schrodinger equation.
This formulation leads to predictions that are unique to
incoherent solitons.
For example, incoherent solitons can have any shape in
their two-dimensional cross-section, and can even travel
in parallel without interacting, unlike coherent beams in
the same intensity dependent medium. Experiments have now
confirmed these predictions. The crucial concept here is
that all these phenomena can be appreciated by simple physics.
Now lets move on to discuss device and logic applications:
especially switching light with light.
The dream of photonics is to have a completely optical
technology. Here the traditional carriers of information,
electrons, are envisaged to be replaced by photons for devices
based on switching and logic. Spatial solitons offer one
potential way to achieve this dream.
We have described the physics of how waveguides are induced
by solitons. The challenge is to develop methods for controllable
steering of these induced waveguides by light itself and
to produce reconfigurable waveguides. But this is easy.
Because as I have shown you, solitons can attract, repel
and guide beams, light can be used to switch light for various
device and logic applications which are presently being
performed by electronics.
Now in one scheme the solitons can be considered as the
information flow itself or the soliton merely induces optical
waveguides which in turn carry the information. This information
can take the form of a weak ("dim") probe beam at a different wavelength or different
polarisation than the ("bright") soliton beam. In either
scheme, intricate virtual circuitry can be written in bulk
nonlinear media. |
I have been describing the simplest form of spatial switching.
Alternatively, it is possible to induce waveguide components
by having two or more solitons interact. Here the solitons
are used to guide dim signal beams. In this way structures
can be created to tap a weak signal, re-route it and this
process can be made both dynamic and reconfigurable. For
example, colliding solitons can induce a waveguide coupler.
And, the amount of coupling can be controlled by changing
the physical properties of one soliton. Finally, it is also
possible for two colliding solitons to fuse, or to split
into two or more solitons - creating additional dimensions
of switching. If two solitons can fuse, it must be also
possible to reverse the process.
A weak signal can be used to split a soliton beam into
two or more beams, allowing for even greater versatility.
Depending on the photosensitive properties of the material,
the circuitry can have a life span which allows for the
possibility of self-reconfigurable circuits. Such plasticity
opens the door to adaptive circuits that can be designed
to transform themselves to the desired application. The
speed required for switching depends on the application.
It can be as slow as seconds for circuit reconfiguration
in network application or as fast as picoseconds for optical
computing.
As I said the steering of one soliton by another soliton
or of a weak signal beam by a soliton forms the simplest
case of spatial switching.
Structures can be created to tap a signal, make a copy
or re-route it and these processes can be made dynamic.
For example, the coupling ratio of a two soliton beam induced
coupler can be adjusted by changing the properties of one
of the solitons. It is also possible for two colliding solitons
to fuse, or to split into two or three solitons and to control
this splitting by a weak probe beam, thus creating additional
forms of spatial switching.
Lastly, let me change gears from light guiding light, to
now discuss how to fabricate designer components using solitons.
We have shown how soliton dynamics can be approached from
the perspective of linear waveguides. Curiously the reverse
is also true.
The phenomenon of soliton dynamics provides a method of
actually fabricating ideal lossless waveguide components
whose design had not even been foreshadowed from our knowledge
of linear waveguides. The concept is elementary.
Solitons are allowed to interact in the appropriate photosensitive
material so that the desired induced waveguide configurations
are then permanently written.
For example, consider the optical device known as the X-junction.
This is one of many building block components used for processing
optical signals. It can be used to mix or split two signals
in any desired proportion. From our knowledge on linear
waveguides, we anticipate some reflection and radiation
loss when light travels along one area but solitons can
undergo lossless collision.
And we can pack many such elements on top of each other.
Because, unless beams collide at grazing incidence, they
always pass through one another without influencing each
other. In this way it is possible to compress circuitry
into a compact space with many circuits sharing the same
physical location.
Furthermore, certain photosensitive materials offer the
potential for erasing one light written device and replacing
it by another. Hence, we have the building blocks for dense,
reconfigurable, virtual circuitry.
We have unfolded the building blocks for virtual circuitry
leading to a transparent cube with a myriad of interconnections
created, maintained, and arranged by light itself - with
truly awesome potential. I have told you some of the things
that solitons can do but will this lead to viable technology? |
I am not the person to answer this question. But I do know
that some formidable challenges remain in crafting the requisite
nonlinear and photosensitive materials. The present situation
regarding light guiding light is reminiscent of the mid
1960's when optical fibre communication was first being
mooted.
That was deemed a great idea, if only glass absorption
could be dramatically reduced - and in a few years it was.
We are definitely faced with a challenge, but if surmounted,
great things are possible. Scientists have been trying to
confine light to artificial boundaries. Here is the opportunity
for light to be the master of its own destiny.
I spoke at the outset of the extraordinary achievements
since Marconi's historic transmission exactly 100 years
ago. What about the next 100 years? Breakthroughs involve
leaps of the mind. If we could somehow accelerate the very
process of such creative leaps, this in turn could accelerate
the pace of technological breakthroughs. And, this possibility
consumes any energies of late.
Now the greatest block to creativity is the simple fact
that we are blinded by our knowledge.
We don't actually see what is out there - rather we can
only interpret the world through our beliefs and knowledge
- our mindsets - accumulated from past experiences. This
is a brilliant strategy for manoeuvring rapidly in familiar
situations, but it is an impediment when confronted with
a novel idea.
Suppose we could bypass our mindsets and see the world
as it is? Suppose we could become, more literal. Certain
brain damaged people do just that. They are literal but
they are so at a cost. They lack the necessary mindsets
to appreciate what they do see.
For example, Nadia, a severely brain damaged three year
old can draw in incredible detail. She did so spontaneously
without training. Yet, Nadia could not recognize her mother
from the nurse and she has no language ability. Nadia also
has no idea how she does it. She just 'sees it'!
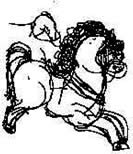
Suppose it were possible to artificially switch off a part
of the brain in normal people, to switch off our mindsets
and see the world as it is? In particular, to switch off
that specific part of the brain which is damaged in Nadia.
In fact, it is possible. We do this by using transcranial
magnetic stimulation - magnetic pulses used to induce artificial
lesions. These magnetic pulses create electric currents
via the Faraday effect which in turn shut down local regions
of the brain. |
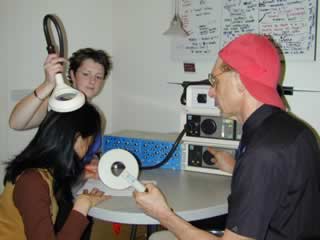
As described by the recent BBC documentary "Fragments
of Genius", we and other groups have been performing
these experiments with very promising results. For example,
people do seem to be able to draw better, more realistically.
We are right now developing EEG assisted feedback control
techniques to enhance objectivity without the need for the
magnetic pulse stimulation. So perhaps, the great technological
achievement of the future will be that which help accelerate
our objectivity.
And, curiously, this new frontier of, lets call it mind
physics, actually involves electromagnetic technologies.
Something that Marconi himself would have appreciated, and
something that Marconi's genius could well have made accessible
to everyone. |
|
|
|
|